Astronomical Site Monitor (ASM) instruments
The ASM instrument suite is critical for our operations. This page contains information about the ASM instruments used in the ESO’s Paranal Observatory:
- Monitoring of meteorological conditions, e.g. temperature, humidity, barometric pressure, and wind speed and direction.
- Monitoring of atmospheric turbulence, e.g. integrated atmospheric column seeing, coherence time of the turbulence, isoplanatic angle, fraction of the integrated turbulence found in the ground-layer (first 250 m above the surface), and vertical profiles of the Cn2 (spatial variance in the air index of refraction at optical wavelength).
- Monitoring of the precipitable water vapour.
- Monitoring of sky transparency condition.
- Monitoring of earthquake activity.
Differential Image Motion Monitor (DIMM)
The Differential Image Motion Monitor (DIMM) is the instrument used for the monitoring of the integrated seeing in at atmospheric column. This type of instrument has been in operations at the ESO Observatories for many decades [1]. The seeing for the Paranal site has been well characterized [4].
Currently, and since April 5th 2016, the DIMM is located on a 7.5 m high platform located away from any disturbing infrastructure.
The DIMM’s seeing is reported in units of arcsecs, normalized to a unity airmass (i.e. as if measured along the zenith direction) and at a wavelength of 500 nm (V band). Note that while the seeing measured with the DIMM is computed at 500 nm (V band) and at zenith, the image quality constraint in the Observing Blocks corresponds to a specified reference wavelength and at the airmass the observations are conducted. The DIMM seeing is recorded in the fits headers under the keywords TEL AMBI FWHM START/END.
The previous tower was located towards the north of the VLT platform and close to the VST telescope. The ASM database includes the seeing record from this station from 1998-08-19T22:57:13 to 2016-04-04T10:08:39.
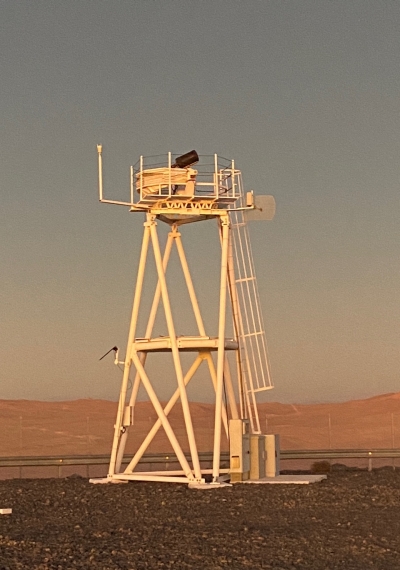
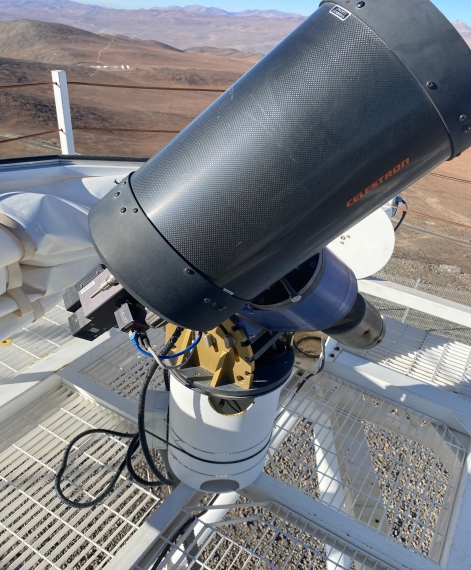
Multi Aperture Scintillation Sensor (MASS)
The expected improvement on the science image quality brought by new adaptive optics facilities (AOF) is strongly dependent on the vertical distribution of the turbulence. The a priori knowledge of this atmospheric parameter is thus a requirement to schedule AOF observations most efficiently. Two dedicated instruments are fulfilling this task.
One is called Multi Aperture Scintillation Sensor [3]. The MASS is coupled to the DIMM and provides information about turbulence profile in the atmosphere [4]. The MASS is working automatically and is running when the DIMM is running. By combining the MASS and DIMM measurements, estimates for the isoplanetic angle (Theta0) and the coherence time (Tau0) are provided in real time (without relying on predictions of the wind at 200 mbar as for the old ASM).
The MASS also provides a seeing estimate in a free atmosphere (above 1 km) that is available in the ASM database. The total seeing (given by the DIMM) and the free-atmosphere seeing (given by the MASS) can be used to have an estimate of the fraction of the turbulence closer to the ground. At the Paranal site, the long-term ground-layer-fraction of the overall turbulence in the vertical atmospheric column is 62%. That is, 38% of the turbulence is confined to the free-atmosphere, mainly in the top of the troposhere closer to the tropopause and is induced by wind-shear in the vicinity of the sub-tropical Jet Stream.
The turbulent surface layer has been documented in the work of Lombardi et al. [5].
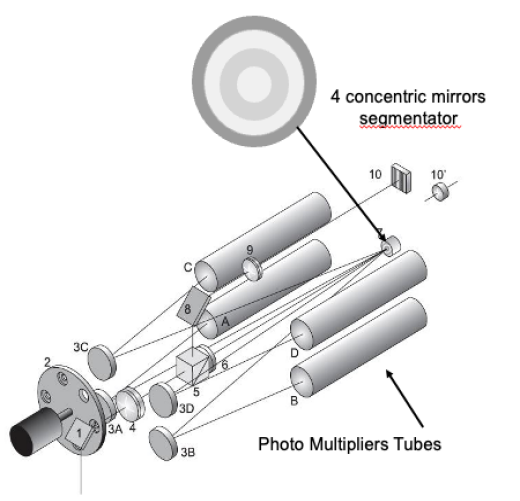
Fig. 3 shows how the light of a bright star is collected by the feeding telescope and enters to the detector box. Upon reflection from the folding mirror 1, it passes through the focal aperture mounted on the disk 2, controlled by the stepper motor. The Fabry lens 4 forms an image of the telescope pupil on the “segmentator” 7 with a de-magnification factor around 20. The segmentator consists of four concentric mirrors with different tilts, each mirror reflecting the beam at a certain angle. The diameter of the inner mirror is 1 mm, the diameter of the outer mirror is 6.5 mm (2 cm and 13 cm, respectively, as projected onto the telescope pupil). The four reflected beams fall on the spherical mirrors 3A-3D which re-image pupil at the photo-cathodes of the PMTs A, B, C, and D. The dichroic beam-splitter 5 reflects the light with wavelengths long-wards of 600 nm to the viewer which consists of the folding mirror 8, the lens 9 and the eyepiece 10’ which can be replaced by a small TV camera 10. Spectral filtering of the detected light is provided by the glass filter 6 glued to the beam-splitter. The segmentator is a non-standard, custom-made optical element. First, four closely matched cylinders were machined from stainless steel and assembled together. Then the working surface was ground and polished at an angle with the cylinder’s axis. The surface is made slightly concave to avoid the divergence of the reflected beams. After aluminization, the cylinders were turned around their common axis and the segmentator was re-assembled in its final configuration.
SLODAR
The Slope Detection and Ranging (SLODAR, [6]) provides turbulence profiles for the ground layer of the atmosphere up to ~1500 m. This instrument uses a crossed beams method based on observations of double stars using a Shack-Hartmann wavefront sensor. The optical turbulence profile (OTP) is recovered from the cross-correlation of the wavefront slope measurements for the two stars [https://jamesosborn.webspace.durham.ac.uk/slodar/].
The use of the SLODAR data is critical for HAWKI+GRAAL and MUSE+GALACSI Wide Field Mode observations.
A typical SLODAR profile is shown in here:
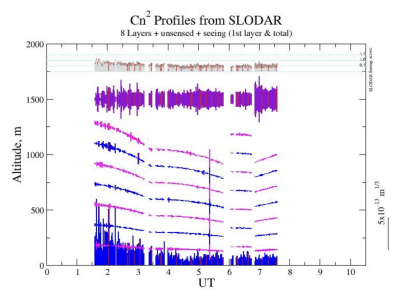
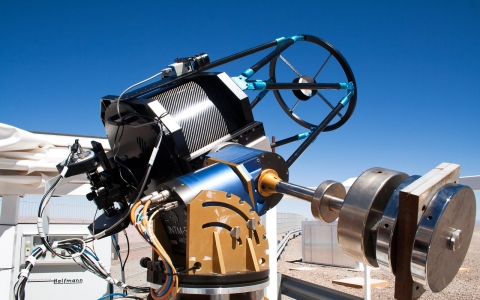
Stereo-SCIDAR
The Stereo Scintillation Detection And Ranging (Stereo-SCIDAR, [7]) is a technique to allow high sensitivity vertical profiles of the atmospheric optical turbulence and wind velocity profile. This instrument uses a stereoscopic system with the scintillation pattern from each star of a double-star target incident on a separate detector (see sketch). At Paranal, the instrument is located in the E0 pad of the VLTI Auxiliary Telescope (AT) configuration, and the double-stars acquisition is done using the AT. The S-SCIDAR is used monthly during full moon nights for the long-term monitoring of the turbulence profile at Paranal, up to about 20 km, with a vertical resolution of typically 200-300 m.
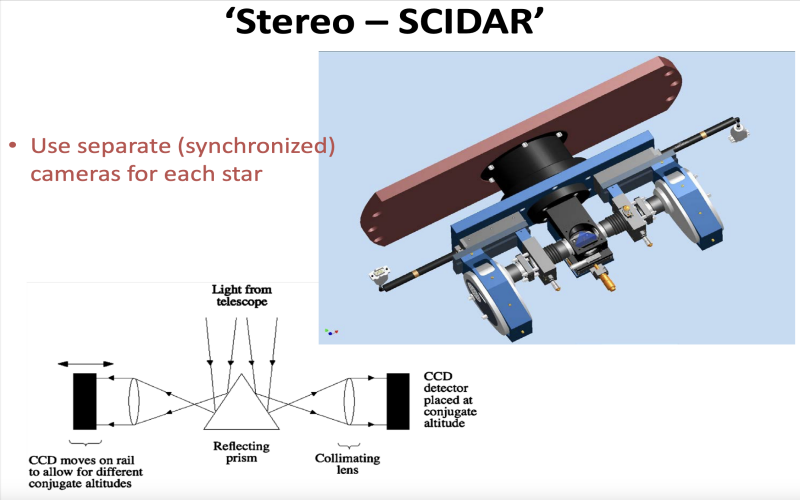
LHATPRO radiometer
The instrument measures the amount of Precipitable Water Vapor (PWV) via probing the atmosphere in two frequency ranges focusing on two prominent emission features: an H20 line (183 GHz) and an O2 band (51-58 GHz), using 6 and 7 channels, respectively. The radiometer retrieves the profile of humidity and temperature up to an altitude of about 12 km [8]. The radiometer is designed and built for continuous operations without human interaction and can be controlled remotely.
An IR sensor is also part of the instrument package, providing measurements of the brightness temperature of the sky down to -100 C. This specific model detects not only water-bearing clouds, which are considerably warmer, but also cold high-altitude clouds. The statistical analysis of the variability in the IR temperature of the sky, measured at 13 pointing directions (6 at 30 degrees elevations, 6 at 60 degrees elevation, 1 at zenith) allows for an estimation of the sky transparency, i.e. whether it is photometric, clear, or whether there are thin or thick cirrus clouds (see plot that shows an example of sky condition at Paranal). The assessment of the sky trasnparency is based on the statistical analysis of IR measurements of the sky in periods of 30 minutes.
The following figure shows an example of PWV time series measured at Paranal.
Presentation on Operational aspects of the LHATPRO radiometers.
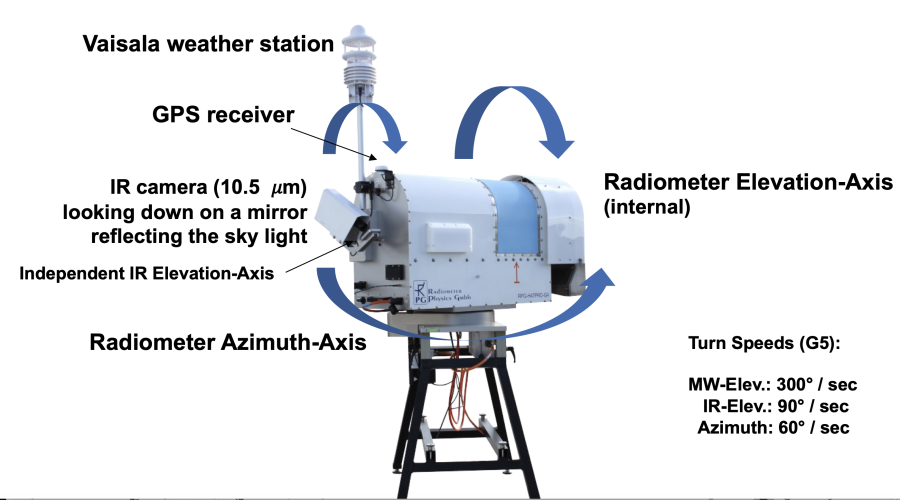
VAISALA
ESO uses weather stations from the VAISALA vendor. These weather stations comprize a set of sensors to monitor wind, rain, temperature, humidity, and barometric pressure [9]. Various of these weather stations are installed in a weather tower at different heights of 2 m, 10 m, 20 m, 30 m above ground. The obtained data is used to support the Telescope Control System by providing relevant information, e.g. temperature and surface barometric pressure, required to compute the atmospheric differential refraction. Temperature, wind, and relative humidity data are essential to support the safety of the operations.
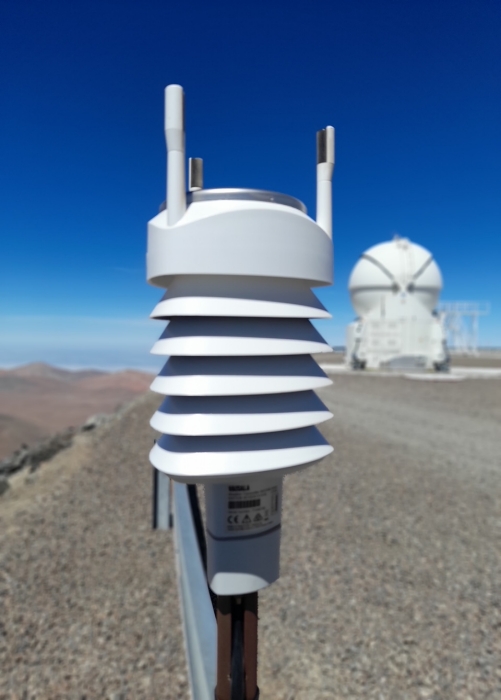
ALPACA
The All Sky Paranal-Apical Camera (ALPACA), located on the platform, allow to monitor the sky transparency during the night.
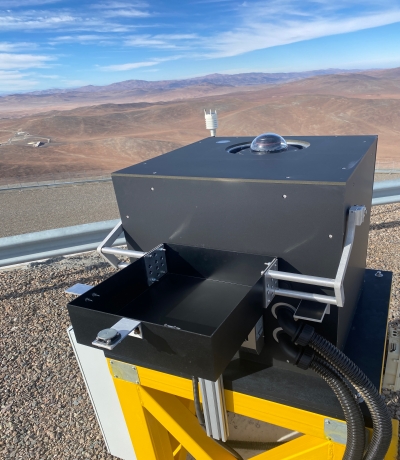
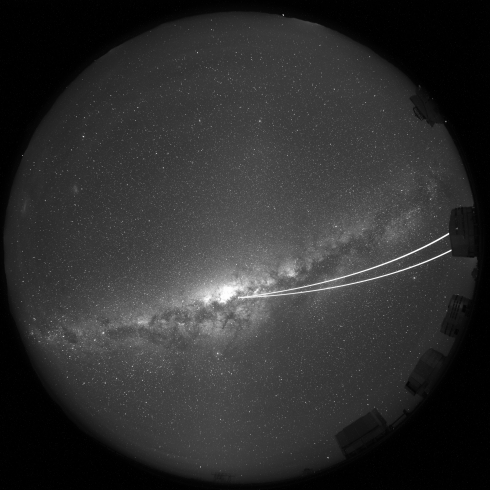
References:
[1] M. Sarazin and F. Roddier, “The ESO differential image motion monitor”, Astron. Astrophys. 227, 294-300 (1990)
[2] Marc Sarazin, Jorge Melnick, Julio Navarrete, Gianluca Lombardi, “Seeing is Believing: New Facts about the Evolution of Seeing on Paranal,”, The Messenger 132 – June 2008
[3] Victor Kornilov, Andrei A. Tokovinin, Olga Vozyakova, Andrei Zaitsev, NicolaiShatsky, Serguei F. Potanin, Marc S. Sarazin, "MASS: a monitor of the vertical turbulence distribution," Proc. SPIE 4839, Adaptive Optical SystemTechnologies II, (7 February 2003); doi: 10.1117/12.457982
[4] Andrei Tokovinin and Victor Kornilov, “Accurate seeing measurements with MASS and DIMM,” Mon. Not. R. Astron. Soc.381,1179–1189 (2007), doi:10.1111/j.1365-2966.2007.12307.x
[5] G. Lombardi, J. Melnick, R. H. Hinojosa Goñi, J. Navarrete, M. Sarazin, A.Berdja, A. Tokovinin, R. Wilson, J. Osborn, T. Butterley, H. Shepherd, G.Lombardi, J. Melnick, R. H. Hinojosa Goñi, J. Navarrete, M. Sarazin, A.Berdja, A. Tokovinin, R. Wilson, J. Osborn, T. Butterley, H. Shepherd, "Surface layer characterization at Paranal Observatory," Proc. SPIE 7733, Ground-based and Airborne Telescopes III, 77334D (6 August 2010); doi:10.1117/12.856772
[6] Richard W. Wilson, “SLODAR: measuring optical turbulence altitude with a Shack-Hartmann wavefront sensor,”, Mon. Not. R. Astron. Soc.337,103–108 (2002)
[7] H. W. Shepherd, J. Osborn, R. W. Wilson, T. Butterley, R. Avila, V. S. Dhillon3and, T. J. Morris, “Stereo-SCIDAR: optical turbulence profiling with high sensitivity using a modified SCIDAR instrument,” MNRAS437,3568–3577 (2014), doi:10.1093/mnras/stt2150
[8] Kerber, F., Rose, T., Chacon, A., Cuevas, O., Czekala, H., Hanuschik, R., Momany, Y., Navarrete, J., Querel, R. R., Smette, A., van den Ancker, M. E., Cure, M., and Naylor, D. A., “A water vapour monitor at Paranal Observatory,” in SPIE Conference Series, 8463N (2012)
[9] https://www.vaisala.com/sites/default/files/documents/WEA-MET-ProductSpotlight-WXT530-B212232EN-A.pdf