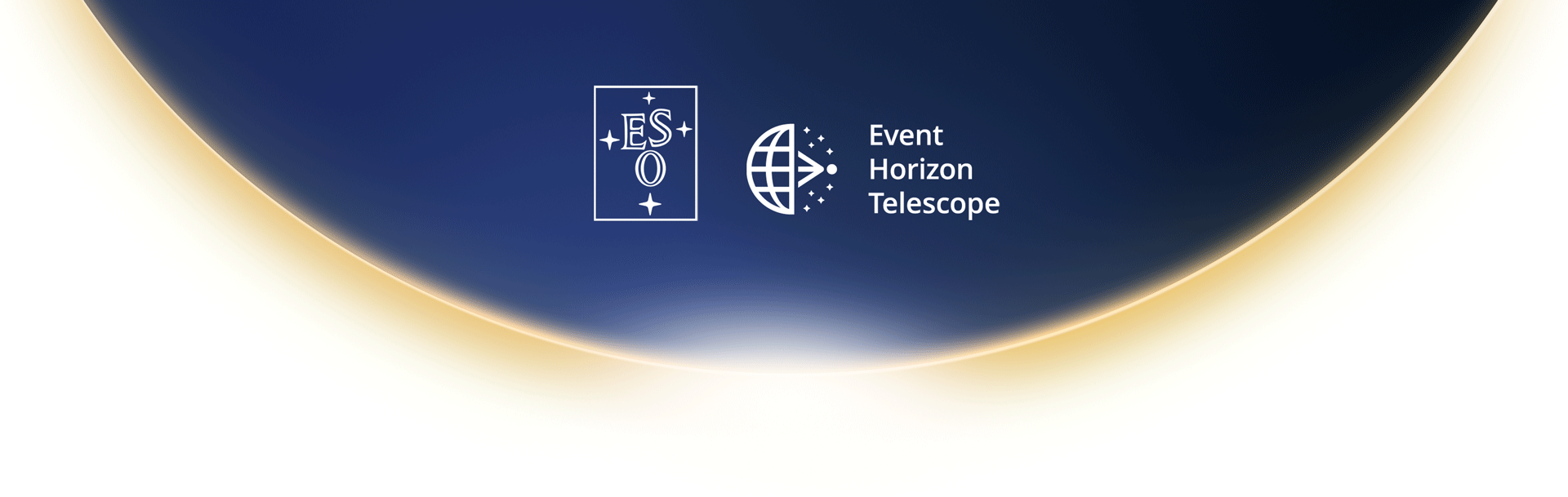
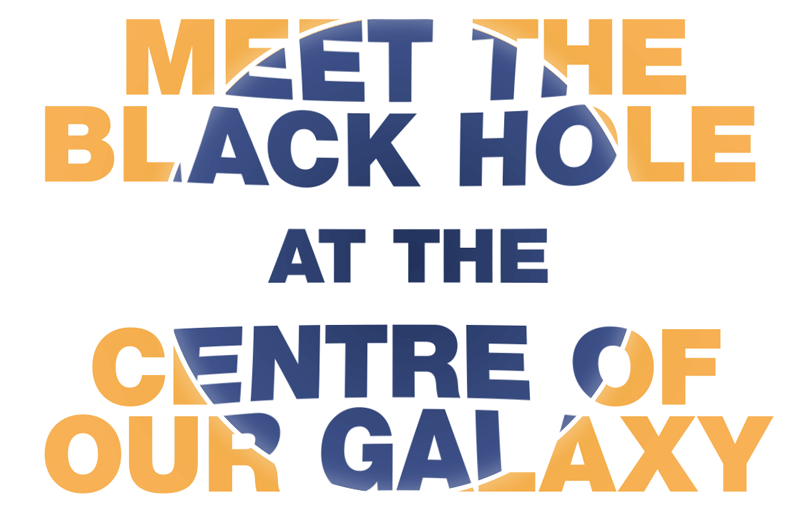
Press Conference and Public Q&A
Watch or rewatch the livestream of the press conference revealing the world’s first image of Sagittarius A*, the supermassive black hole at the heart of our galaxy, the Milky Way. This groundbreaking image was taken by the Event Horizon Telescope (EHT), a network of radio telescopes spread across the Earth. The image was unveiled at simultaneous press conferences around the world, with ESO hosting the European event at its headquarters in Garching, Germany.
Continue watching after the press conference (from 16:30 CEST) to see scientists from the EHT Collaboration answer questions from the public about the science and technology behind the image. If you are watching live and would like to participate, you can either send us your questions through our YouTube channel or on Twitter using the hashtag #askEHTeu.
The Image
Today, the EHT—composed of the Atacama Large Millimetre/submillimeter Array (ALMA), the Atacama Pathfinder EXperiment (APEX) and other radio telescopes—has taken the first ever image of Sagittarius A* (also known as Sgr A* so ‘sadge-ay star’), the supermassive black hole at the heart of our own galaxy. Although black holes cannot be imaged directly as they are entirely dark, luminous gas around them reveals a telltale signature: a central dark feature or "shadow" surrounded by a bright ring. It was this signature that the EHT was able to capture.
This truly astonishing feat has allowed us to peer into our galaxy’s hidden heart, bringing us ever closer to understanding our place in the cosmos. The image provides the first ever direct visual evidence of the black hole at the centre of the Milky Way.
The image is only the second ever taken of a black hole, after the EHT revealed the image of the supermassive black hole in the Messier 87 (M87) galaxy in 2019. This image has since gone on to gain household fame, being featured in media outlets worldwide.
Press Release
The EHT Collaboration is a global network of over 300 researchers from 80 institutes around the world. Their cooperation has enabled this incredible image of Sagittarius A* to be obtained. This press release, written in a collaboration between science communicators and EHT scientists, explains the story behind this stunning research. It's available in multiple languages and includes multiple images and videos.
Sagittarius A*
In 1974, astronomers Bruce Balick and Robert Brown discovered a bright source of radio waves in constellation of Sagittarius at the centre of the Milky Way. At the time, the astronomy community did not know what was causing this loud burst of energy, but Balick deemed the discovery “exciting”, naming the mysterious object Sagittarius A*.
Decades of detailed observations monitoring the motion of stars orbiting Sagittarius A* showed that this bright object was so massive and so compact that it could only be a black hole. Germany’s Reinhard Genzel and America’s Andrea Ghez were awarded a Nobel Prize in Physics in 2020 for this discovery. Ghez used the Keck Telescopes in the US for her team’s research, while Genzel and his team used ESO’s telescopes in Chile. They started their observations using ESO’s New Technology Telescope (NTT), following up with the sensitive instruments on ESO’s Very Large Telescope (VLT) and, more recently, ESO’s VLT Interferometer.

Now, alongside decades worth of studies predicting that Sagittarius A* is a supermassive black hole, the new EHT image provides the first direct visual evidence of the giant at the heart of our galaxy.

Imaging a Black Hole
What does it take to image a black hole? The EHT uses a technique called very-long-baseline interferometry (VLBI) which exploits the Earth’s rotation to form one huge, Earth-sized telescope. The EHT network currently comprises 11 telescopes, though only 8 were used for the first Sgr A* observations. Find out more about how the EHT works in the explainer video below.
The Facilities
Capturing an image of a black hole such as Sagittarius A* requires a huge effort. Though it is our nearest supermassive black hole, Sagittarius A* is still 27,000 light-years away. This means that despite its supermassive size, from Earth, it looks no bigger than a doughnut would on the surface of the moon.
To be able to see an object like this, astronomers require telescopes with high resolution. This resolution is set by the distance between any two observing telescopes in the EHT network: the greater the distance between them, the higher the resolution. This is why the EHT telescope array, which included the 8 facilities below when it observed Sgr A* in 2017, is spread across the globe, from Antarctica to North America, Europe, and South America. Two telescopes that form part of the EHT are APEX and ALMA, co-owned by ESO and operated from Chile’s Atacama Desert.
Once the EHT has finished observing, the data collected must be calibrated and combined. This is no trivial task. Each of the EHT telescopes produces a huge amount of data: hundreds of terabytes per day. This is stored on high-performance helium-filled hard drives and flown to supercomputers at the Max Planck Institute for Radio Astronomy (Garching, Germany) and MIT Haystack Observatory (Massachusetts, USA) for analysis.
It is only after this data is cleaned and combined that astronomers can start using computational tools to extract an image.
Extracting the Image
As their name suggests, black holes are completely dark, making direct imaging of them impossible. The gravity of a black hole is so strong that beyond its event horizon, time and space are distorted. The event horizon is the point of no return around a black hole, beyond which the pull of gravity is so strong that even light cannot escape. It was from this that the name “Event Horizon Telescope” arose.
To capture an image of a black hole, scientists look at the glowing gas and dust that surrounds it. Imaging Sgr A* posed a particularly difficult challenge for astronomers because the clouds of gas surrounding it move extremely quickly, at nearly the speed of light, completing a single orbit of Sgr A* in mere minutes. This means the brightness and pattern of the gas around Sgr A* was changing rapidly as the EHT Collaboration was observing it — a bit like trying to take a clear picture of a puppy quickly chasing its tail.
The 8 telescopes used together to image Sgr A* collected data for many hours in a row, like using a long exposure on a camera. The information obtained was processed using powerful algorithms to reconstruct the appearance of Sgr A*, as well as new tools to account for the quick movement of gas around the black hole.
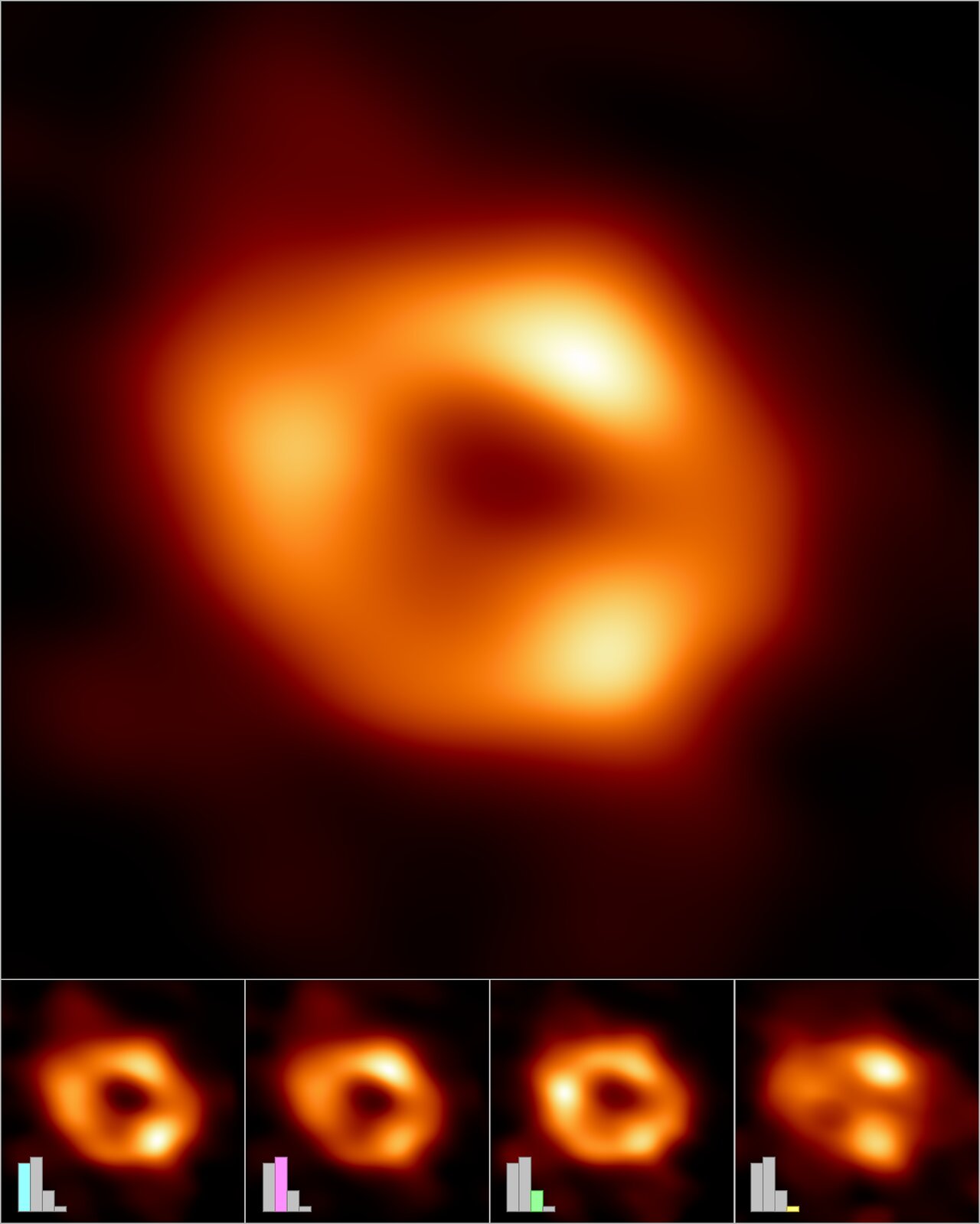
The information from each telescope provides a piece in the jigsaw puzzle of the black hole's appearance. For each puzzle piece, the final image becomes easier to see. Think of it as a giant game of Guess Who — to begin with, astronomers have no information and there are infinitely many ways that the black hole could look. Astronomers can use observations from telescopes like the EHT to narrow down the options and home in on a better guess. With just eight telescopes, the number of possible candidate images is still large. But not every guess is equally likely. Astronomers use a combination of mathematics, physics and sophisticated analytical tools to narrow down the options. In the case of Sgr A*, the EHT Collaboration extracted several images that were averaged to reveal the giant lurking at our galactic centre.
Two Instead of One

Scientists are particularly excited to have imaged not one, but two black holes of very different sizes. The first image produced by the EHT Collaboration was of the supermassive black hole at the centre of the Messier 87 (M87) galaxy, located 55 million light years from Earth. M87’s black hole is far larger than Sagittarius A*. Where Sgr A* has a mass roughly four million times the Sun’s mass, M87's black hole weighs in at 1700 times this figure, making it one of the most massive known black holes. But because the M87 black hole is further away than Sgr A*, the two black holes appear to have roughly the same size in the sky.
Scientists have already begun to study how Sgr A* and M87* compare and contrast to better understand the science of black holes.
The Zoom
This video begins with a view of ALMA, one of the constituent telescopes of the ELT, and zooms in on the heart of the Milky Way, taking us into the depths of the constellation of Sagittarius, 27,000 light years away. On the journey, you can see some of the increasingly detailed pictures we have taken of the galactic centre, including those of stars moving around the invisible compact object known as Sgr A* taken using ESO telescopes. Finally, you will see the EHT Collaboration’s image of the black hole.
Blog Posts
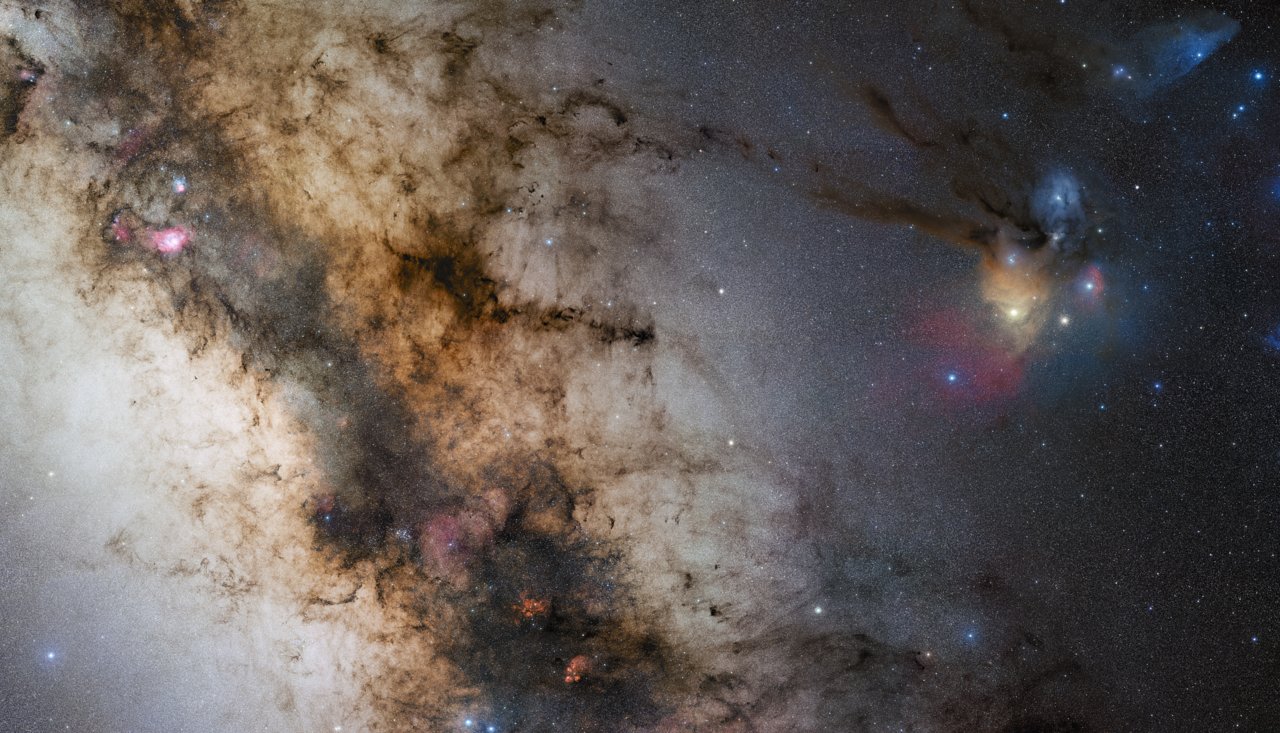
The story of our quest for Sagittarius A*, the supermassive black hole at the heart of the Milky Way
This blog post tells the decades-long story of how astronomers have cornered the black hole lurking at the centre of our galaxy. From the first radio detections in the 1930s to the more recent observations of stars orbiting the black hole with ESO’s VLT and other telescopes, the article describes the unique technology required to zoom in ever closer to our galaxy’s centre.

Spot the difference: Imaging Sagittarius A* and M87*
The challenges of taking the first image of the Milky Way’s dark heart
The new image of the Milky Way’s black hole, Sgr A*, released by the Event Horizon Telescope looks remarkably similar to that of the black hole in the M87 galaxy, yet it posed unique challenges. In this blog post three EHT experts –– Katie Bouman, Kazunori Akiyama and José L. Gómez –– tell us in detail what made it so difficult to image Sgr A* and how they overcame these problems.
The Visuals
Frequently Asked Questions
Most of the answers provided here are modified from originals published in the Event Horizon Telescope Collaboration, and are courtesy of the EHT Outreach Committee. We recommend checking the FAQ section on their website for more answers!
Are there more black holes, other than Sgr A*, in our Galaxy?
It is predicted that many smaller black holes, called stellar-mass black holes, exist throughout any galaxy. They are remnants of massive stars that have exploded as supernovae. The EHT studies the supermassive black holes Sgr A* and M87* because their apparent sizes are much larger than those of stellar-mass black holes when viewed from the Earth, so they are easier to image.
The EHT used eight telescopes for a week-long observation of black holes in April 2017. Which telescopes were used, and was it difficult to get so much observing time on all these telescopes?
The EHT Collaboration consider themselves very fortunate that their science is widely viewed as compelling, and that many observatories supported the EHT observations. The following radio telescope observatories were involved in the 2017 observations: Atacama Large Millimeter/Submillimeter Array, Chile, Chajnantur Plateau), (Atacama Pathfinder Experiment, Chile, Chajnantur Plateau), IRAM 30m (Institute de RadioAstonomie Millimtrique, Pico Veleta, Spain), LMT, (Large Millimeter Telescope, Mexico), SMT (Submillimeter Telescope, US, Arizona), JCMT (James Clerk Maxwell Telescope, US, Hawaii), SMA (SubMillimeter Array, US, Hawaii), SPT (South Pole Telescope, South Pole).
Are there any smaller black holes near the Solar System and can you detect them by using the EHT?
There are lots of stellar-mass black holes that are much closer to the Solar System than Sgr A*. However, the size of a black hole is proportional to its mass, so these stellar-mass black holes look much smaller than Sgr A*. Even if we can detect some stellar-mass black holes with the EHT, we would not be able to resolve the emission around them, on the scale of their event horizon.
The closest currently known black hole is V616 Monocerotis, 3,000 light-years away, with a mass of 11 times our Sun (Sun’s mass, or Solar mass, is approximately 333 000 times the mass of the Earth). It orbits a star half the size of the Sun with a period of about 8 hours, which causes its light to vary periodically. At double the distance, we have Cygnus X-1 (15 solar masses), orbiting a star of 30 Solar masses with a period of around 6 days.
A black hole, by definition, does not emit radiation. In fact, it captures everything that falls onto it. So how do you observe something that does not radiate?
It is true that a black hole itself does not emit light. However, the EHT observes the nearby surroundings of a black hole. The gas that surrounds the black hole does in fact radiate, so by observing this region, the EHT may observe structures that result from the strong gravity of the black hole.
Can we really photograph a black hole? Are they not entirely dark, since no light can escape them?
The first image of a black hole is not a classical photograph. It is a radiolight image, the result of complex observational and computational interpretation. Further, it is not of the black hole itself, but of the "shadow"—the closest we can come to imaging a completely dark object that consumes all light and matter. The black hole boundary—the event horizon for which the EHT is named—casts this shadow. General Relativity says the superheated material around the black hole will glow and illuminate the strongly warped region of spacetime, making it visible to interferometer observation and measurement.
This animation and this article may help in better understanding how the image of a black hole is formed.
What if the black hole shadow is oblate (flattened at the poles) or prolate (elongated between the poles), does it mean that there is something wrong with the General Theory of Relativity?
Finding something contrary to our expectations (some of which you may find in the EHT simulations gallery) would certainly be interesting. It would not necessarily mean that the General Theory of Relativity is wrong, but it would imply that we still have more physics to understand. Discoveries of gravitational waves from merging black holes by LIGO and collaborators have recently confirmed one of the most fundamental predictions of this theory.
What is very long baseline interferometry, the technique used by the Event Horizon Telescope?
Very-Long-Baseline Interferometry (VLBI) combines the signals collected by radio telescopes located hundreds or thousands of kilometers apart, stretching the baselines to the maximum lengths possible on Earth to create a “virtual” telescope the size of the whole planet.
This increases the resolution achieved by radio interferometers, such as ALMA, by hundreds of times. This enables astronomers to view the cosmos in sharper detail to such extremes as being able to look at the shadow of the supermassive black holes lurking at the heart of galaxies. This is the ultimate goal of the EHT. The primary targets of the EHT are the two largest supermassive black holes as seen from Earth: Sgr A*, the Milky Way's black hole, and M87*, the black hole in the core of the M87 galaxy. The EHT network looks at the radio waves emitted by the disc of gas around the event horizon of the two black holes.
As the EHT antennas are spread across continents, their signals cannot be combined and analysed on site, like in ALMA. Instead, the signals must be recorded and brought together to be analysed after the observations have been performed.
With the EHT stretching across the whole planet, have we reached the longest possible baselines, hence creating a limit to how sharp we can look at the cosmos? The answer is no, as space-based VLBI is already operating with radio satellites offering even longer baselines than the size of the Earth.
Our planet will not be the ultimate frontier of interferometry but simply the beginning.
Will the EHT take razor-sharp images of the event horizon?
Obtaining sharp images of a black hole event horizon is very challenging and the EHT will do its best to produce the sharpest images ever obtained. The quality of the images depends on the arrangement of the telescope array, weather conditions at the telescope sites, as well as blurring of images as the light travels from the black hole toward the Earth. Speaking of razor-sharp, here is an interesting calculation. A razor blade edge is typically 400 nanometers wide — less than a millionth of a meter, or roughly one sixty-thousandth of an inch. Held at arm's length, the angular size of a razor blade edge is approximately half an arcsecond. The resolution of the EHT is more than a million times better than that! To get a rough idea of how much better that is, imagine counting individual dimples on a golf ball in Los Angeles... from New York.
How much data is recorded during an observation and how it is transferred to the central processing facilities?
Depending on instrument setup, weather, position within the array, and other factors, an EHT five-day observing campaign can produce about a Petabyte (PB) of raw data per observatory or a thousand billion bytes. For example, the total amount of raw data recorded in April 2017 was about 3.5 PB. This data must be recorded to hard disks and manually transported to central processing facilities in Germany and the United States, as transferring it via the Internet would take considerable time. Approximately 5.5 PB data were recorded during the April 2018 observations. Future campaigns are expected to record up to 15 PB per year.
Data storage and processing enthusiasts may want to look up more details in this article.
Why is it important to study black holes? What is there to be learned from the EHT observations?
As a scientific collaboration, the aim of the EHT is not only to prove the existence of black holes, but also to understand the physics of black holes and their surrounding environments. There is ample indirect evidence from various astronomical studies indicating that black holes exist, including the investigation of nearby objects that are subjected to the gravitational pull of a black hole. These are circumstances well explained by Einstein’s General Theory of Relativity. It was the direct observation of the immediate environment surrounding a black hole—the event horizon—that had never been achieved, until now. With the release of the first results in April 2019, the EHT has filled a significant gap in our knowledge.
Black holes are laboratories for testing fundamental theories that explain how the Universe works on the largest and the smallest scales. Physicists currently do not understand how to create a single physical theory that works both on extremely large and extremely small scales and, hence, explain the physics of black holes in detail. With the EHT results, scientists are able to directly probe the behaviour of spacetime at the black hole boundary.
In addition to the physical theories, there are many details of plasma physics that are not completely understood. Properties of the hot gas surrounding and being pulled into the black hole beyond the event horizon are not fully known. But, understanding these properties is crucial for interpreting black hole images because it is this glowing plasma that produces radiation captured radio telescope arrays. This glowing hot gas illuminates the shape of spacetime around the black hole and EHT observations will help to better understand the properties and behaviour of these extreme environments.
How realistic are movie depictions of black holes, for example in the "Interstellar"?
The portrayal of the black hole "Gargantua" in the movie Interstellar is somewhat realistic, in that they show the general shape of these cosmic structures accurately. However, for aesthetic reasons, the movie producers removed one important aspect of the imaging to make the black hole more dramatic: the Doppler effect. In reality, the approaching side of the rotating accretion disk would appear brighter and hotter (blueish white), while the receding side would be dimmer and more red. Although the Interstellar production team consulted with physicists (most notably, Nobel-prize winner Kip Thorne) and based Gargantua on mathematical simulations, the movie ultimately showed a more artistic—brighter and more symmetric—rendering of the black hole. Furthermore, "Gargantua" is shown to have a razor-thin accretion disk, whereas astronomical observations of both Sgr A* and M87* indicate that their accretion discs are much thicker, with a more doughnut-like appearance.
Educational Resources from the ESO Supernova
The ESO Supernova Planetarium & Visitor Centre has educational resources on its (online and on-site) exhibition related to the Milky Way and black holes. A few examples include:
Panel 1004: What does the Milky Way look like?
Panel 1010: What lurks at the centre of the Milky Way?