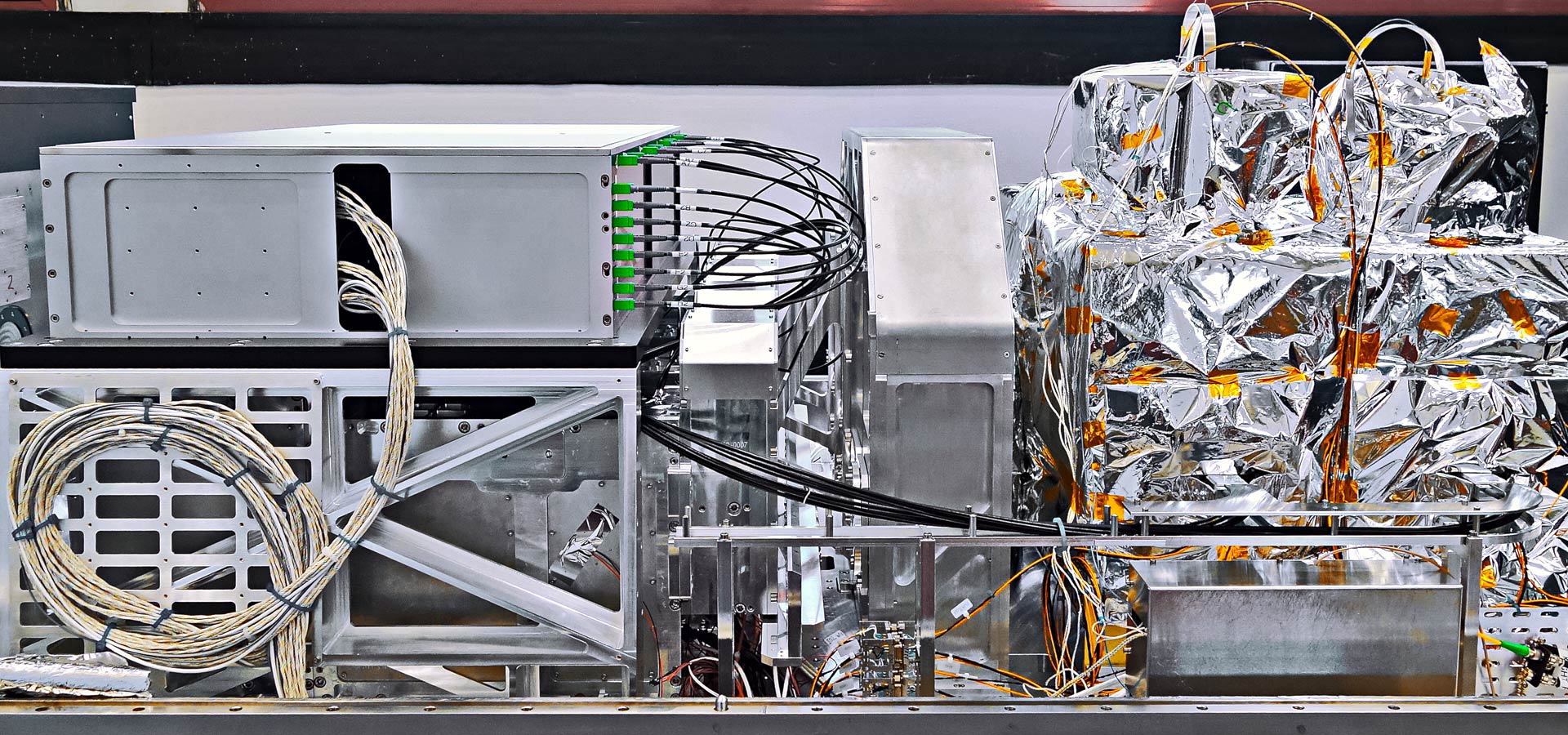
Advancing Technology for Galactic Observations
Frank Eisenhauer discusses observing a close approach at the galactic centre
- The technology behind the observation of general relativistic effects at the galactic centre
- Using adaptive optics and interferometry for highly precise infrared observations
Q: Can you tell us a bit about what observations your team aimed to make about the galactic centre?
A: We knew that the star S2 would make a close flyby of the black hole in the centre of the Milky Way in 2018, making it possible to study the effects predicted by Einstein’s theory of general relativity. We expected the motion of the star to deviate from a Keplerian orbit based on Newton’s laws. Our observations aimed to see these differences, so, to see any visible changes, we had to improve our observation accuracy by several orders of magnitude compared to previous measurements.
Q: What did you and your team do to prepare for this year’s observations?
A: Our instrument — GRAVITY — was finished about three years ago and arrived in Chile, the site of ESO’s telescopes. Then the most intense period started: making GRAVITY work together with all four telescopes of the Very Large Telescope (VLT). In the summer of 2016, we had our first observations of the galactic centre, which, for the first time, showed not only S2 but also the black hole with unprecedented resolution. Since then they have become our faithful companions whenever we visit for a look. For the closest approach in 2018, the team returned for further observations every month.
Q: What kind of telescope did the team use to observe the galactic centre?
A: So, we needed a “super-telescope”, which we created by combining the four largest ESO telescopes of the Very Large Telescope (VLT) with a technique called interferometry. This technique is well established in radio astronomy, i.e. when observing at longer wavelengths, but many thought it would be impossible to achieve that level of sensitivity and accuracy at infrared wavelengths. And, yes, it was not easy. But the star would not wait for us — and in the end, we were ready in time!
Q: Can you explain how infrared interferometry works?
A: With an interferometer, you combine the light received from different telescopes. The big challenge is to combine this light properly, or “in phase” as the physicists say. This means that the optical path lengths between the four telescopes and our instrument have to be controlled with the precision of a fraction of the wavelength—several hundred times smaller than the thickness of a human hair—while the telescopes are separated by as much as 130 metres.
Q: Can you tell us a bit about the ESO instruments used and the differences between them?
A: The group around Reinhard Genzel at the Max Planck Institute for Extraterrestrial Physics (MPE) started to observe the galactic centre with the SHARP I camera on the New Technology Telescope in the 1990s. At that time, high-quality infrared detectors became available and we were the first to use them to peer through the dust cloud obscuring the galactic centre. Then came NACO with the VLT, which lead to the breakthrough with the first orbit measurement in 2002. In parallel, we developed SINFONI, the first near-infrared imaging spectrograph for the VLT, which has given us crucial velocity measurements since its installation in 2003. And, finally, GRAVITY now combines all four VLT telescope to a “130-m super-telescope.”
Q: What about these instruments makes it possible to see through the curtain of dust and stars?
A: The galactic centre is hidden behind dense dusts clouds — but this is only true for visible light. If you go to infrared wavelengths, you can see through to the stars beyond. However, part of this radiation is absorbed by the Earth's atmosphere, and in particular water vapour in the air. This meant we needed to go to a high place, with less atmosphere above us, and a dry place, i.e. a desert. This is why the Paranal observatory, on top of a high mountain in the Atacama desert, was the perfect place for these observations. But even for the best observing conditions, the atmosphere’s turbulence blurs the images, which is why we need adaptive optics (AO).
Q: Can you tell us a bit about the Adaptive Optics system for IR imaging? Are there any challenges using AO to observe the Galactic Centre?
A: Adaptive optics means that you measure the distortion from the Earth’s atmosphere a few hundred times per second, and correct it in real time with a deformable mirror. For this, you need a bright reference star. Unfortunately, there are no really bright stars at visible wavelengths close to the galactic centre to measure these distortions. Therefore you have two options: create your own artificial star with a laser beacon, as done with SINFONI, or build an infrared wavefront sensor as we did for GRAVITY.
Q: What advancements in observing technology have made it possible for us to study the galactic centre as compared to 20 or 25 years ago when observations of the galactic centre were really becoming possible?
A: When the group at MPE started observing the galactic centre in the 1990s, we could observe with 150 milliarcsecond resolution. This is about the angle under which a stadium 300 metres diameter would appear on the Moon. Now, with GRAVITY, we can observe with a resolution as good as 2 milliarcseconds, and measure the separation between the star S2 and the black hole with a precision of just a few tens of microarcseconds. The latter is equivalent to observing two objects on the Moon that are separated by the length of a pencil.
Q: What do you find most exciting about studying the galactic centre?
A: To see so many miracles predicted by the general theory of relativity all in one place: the black hole, stars moving at incredible speed, the time dilation, and many other phenomena. The centre of the Milky Way is and will remain our Rosetta stone for deciphering these wonders.
Numbers in this article
2 | Highest resolution of GRAVITY observations in milliarcseconds. |
130 | Effective size of the “super telescope” when VLT telescopes are combined in metres. |
2018 | The year S2 made the latest close fly-by the SgrA*. |
Links
- Research Paper: “Detection of the Gravitational Redshift in the Orbit of the Star S2 near the Galactic Centre Massive Black Hole“, by the GRAVITY Collaboration, to appear in the journal Astronomy & Astrophysics
- Preprint of paper in A&A
- Read the first blog post in the series “Eyeing the Centre of the Milky Way” with MPE Scientist Stefan Gillessen
- Press release
- Max Planck Institute of Extraterrestrial Physics (MPE) Galactic Centre Research Group
Biography Frank Eisenhauer
Frank Eisenhauer is a senior staff scientist at the Max Planck Institute for Extraterrestrial Physics (MPE) in Garching, Germany. He is the Principal Investigator for the GRAVITY and SINFONI instruments, which are currently in use at ESO’s Very Large Telescope in Chile, and has greatly contributed to current understanding of the supermassive black hole at the galactic centre.