SAM - Sparse Aperture Interferometric Masks
Introduction
As part of the original design of the CONICA camera, provision was made for the possibility of utilizing aperture masking interferometry in order to obtain the very highest angular resolutions at the diffraction limit.
In March 2008 this mode was succesfully commissioned and it offered as of p82; the polarimetric flavor of this mode is about to be commissioned in March 2009. Further news will be addedas soon as the commissioning results are available.
Sparse Aperture Masking is useful for very narrow fields of view (theouter limit is set by the resolution of the shortest baseline in themask). Any advantages it enjoys over conventional full-pupil imagingare only manifest at such very high resolutions typically withinseveral resolution elements of the PSF core. In the infrared, thistypically means that the scientific niche is for objects where theentire field of interest lies within several hundred milli-arcsecs froma bright star. (Although there may be ways to mosaic larger fieldstogether, these have never been successfully demonstrated).
Key strengths of a dilute and (ideally) non-redundant pupil are in the mitigation of atmospheric phase noise (seeing) and the use of robust, self-calibrating observables such as the Closure Phase.
Masking is furthermore (by its nature) limited to brighter classes of targets. This is because it is only effective at combating atmospheric phase noise, seeing, and it is counterproductive in photon-starved regimes where detector readout noise dominates.
With NaCo we estimate that the useful magnitude limit for some types of observations could be as faint as 10-12th mag, depending on the level of correction obtained.
SAM must be combined with pupil tracking mode in a way which is transparent to the users.
SAM can be combined with cube mode. This setup is highly recommended to take advantage of the furtherimage enhancing that can be obtained with shift+add data reduction techniques.
For P83 it is hoped to add the SAMPol mode, which adds the use of the Wollaston to perform SAM with polarimetry observations.
Given its complexity, SAM will be offered in VM mode only.
Characteristics
The following text was part of the by the 1st commissioning run in Feb. 2007. The SAM team is composed by Peter Tuthill and Sylvestre Lacour of the School of Physics, Sydney University.
SAM uses special aperture masks in the pupil wheel to obtain the very highest angular resolution at the diffraction limit. There are currently 4 masks available on CONICA, with different characteristics (i.e. number of holes and configuration).
When used correctly, these masks transform the single 8-m telescope pupil into a sparse interferometer array, and it is therefore necessary to understand the principles of optical interferometry and in particular the recovery of complex Fourier data (amplitudes and phases) from the Fizeau interference patterns that result. A full explanation of the mathematical techniques necessary to do this task is beyond the scope of the present document. The interested users are advised to consult sources form the open literature concerning aperture masking..
In brief, masking is useful for very narrow fields of view (the outer limit is set by the resolution of the shortest baseline in the mask). Any advantages it enjoys over conventional full-pupil imaging are only manifest at such very high resolutions Ð typically within several resolution elements of the PSF core. Dynamic ranges obtained within this realm have been demonstrated to be in excess of 200:1 for point source detections. To attain this level of precision, careful analysis of closure phase signals is required and exhaustive understanding of error sources such as PSF calibration and chromatic effects arising from atmospheric dispersion. Furthermore, with full recovery of closure phase signals, complex and arbitrary flux distributions can be mapped with high fidelity. The particular strengths of aperture masking are for relatively bright targets where there is resolved or partially resolved structure within a few resolution elements of bright PSF cores.
The range of masks installed in the camera is intended to span a variety of target fluxes, with the 18-holes mask being tailored to give the best results for bright targets, through to the 7-holes which is for use on the faintest targets.
The design of aperture masks for a telescope needs to take account of several complicating factors. For a given observation, there are trade-offs between various parameters, which means that a range of different masks can be used in order to tailor the experiment to somewhat varying targets and science. The relevant factors to mask design include:
- The desired Fourier coverage (specifically the shortest & longest baseline required)
- The bandwidth of the optical passband to be used for observations
- The apparent brightness of the target star
- The readout noise properties of the detector
- The degree of correction provided by the AO system.
In order to span a promising range of observational parameter space, four masks will be offered with NaCo [Fig. 1]
From left to right: 18holes, 9holes, BB_9holes, 7holes .
In general, the more holes appear in the mask, then the smaller the holes must be (to preserve non-redundancy) and consequently the less light that is passed by the mask. The mask to the left shows the 18 holes configuration which yields excellent Fourier coverage, but which does not pass a large fraction of the incident light. In order to access successively fainter targets, the 9 and 7 hole configurations may be used, although the Fourier coverage becomes markedly worse. There are two different 9-hole configurations: 9holes and BB_9 holes. The distinction between these two being that the simple 9holes offers superior Fourier coverage and slightly higher throughput, but is not suitable for large fractional bandwidth observations. For bandwidths wider than about 10%, the 9 holes mask is unsuited and the BB_9holesshould be used.
The two-dimensional layout of the holes specifies the Fourier coverage afforded by the given mask.
A scaled illustration depicting the optical effect of the masks as projected onto the correctly scaled VLT telescope pupil (assuming ideal optical alignment) is given in Figure 2 below. The large circumscribed circle represents the outline of the VLT primary mirror, while the smaller centered circle shows the silhouette of the secondary mirror. It is important to note that the spiders which support the secondary mirror are not depicted here, but they have an important effect which will be discussed later.
![]() |
![]() |
![]() |
![]() |
Figure 2: Optical diagrams showing the effect of apodizing the pupil with the four 2-dimensional masks implemented in the CONICA camera. 18 holes (top left), 9 holes (top right), BB 9 holes (bottom left) nad 7 holes (bottom right). |
Complete description of the mask and the information needed to calculate the u-v coverage are given in section U-V coverage below.
Measured performance on sky
During the first commissioning run on 17 Feb 2007, the sky was clear. The seeing was however bad, averaging 1.5 arcsec in the beginning, and going over 4 arcsec in the end of the night. The wind speed averaged 15 m/s. Considering we were trying to do high angular resolution observations, this was overall bad conditions. The following results are therefore to be taken as lower limits expectations. An additional commissioning run is foreseen for the middle of March, 2008. News results and information will be posted on the web as soon as they became available.
AB Dor in K and H
AB Dor was observed between 1h17 and 1h42UT. HD41371 was used for calibration and was observed between 1h54 and 2h12UT. For each one of these targets, the data consist of two data cubes in each band (2.24 μm and 1.75 μm). The cubes are sets of 100 exposures of 2 seconds integration time using a 512x512 windowing of the detector. Seeing was around 1.5 arcsec, but AO correction was nevertheless stable, with occasional disruptions. We used the 9 holes mask.
Correction for dark, flat field and bad pixels was applied to our data. An important step was to eliminate exposures were AO correction was unstable. The frequency components (visibilities and closure phases) are then derived. A binary system is adjusted to the data, and the likelihood computed. Figure 3 represent the likelihood for the presence of a binary companion as a function of its relative position to the star. A good fit was obtained for several different positions. This is due to a regular sampling of the u-v plane. Because the minimum spacing between two holes is 1.73 meters, images are obtained with a modulo 1.73/λ rad-1. This corresponds to 208 mas in H and 267 mas in K.
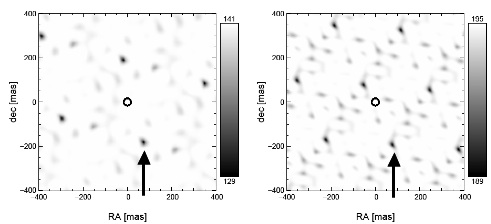
Figure 3: Likelihood for the presence of a secondary star as a function of its position. At maximum likelihood, the flux ratio between the main star and its companion is 1.29±0.14% in K band (left) and 1.47±0.24% in H band (right)
By using data from the two spectral bands, it is therefore possible to identify the position of the secondary star. The position is indicated by the two arrows in Fig. 3. Data fitting also allows deriving the flux ratio between the star and its companion. These results are summarised in the following table:
Star | ABDor | ABDor | HD39213 |
---|---|---|---|
Wavelength | K | H | K |
RA [mas] | 183±6 | -192±9 | 19 ±8 |
DEC [mas] | 75±6 | 77 ±8 | -56±8 |
Relative flux [%] | 1.29±0.14 | 1.47 ±0.24 | 1.22±0.19 |
Delta mag | -4.71±0.15 | -4.58 ± 0.2 | -4.78 ± 0.17 |
Our results on AB Dor are in agreement with the results obtained by coronagraphic means. Sources of potential errors are: 1) Uncertainty on the orientation on the field of view on the pupil. Aperture masking requires freezing the spider arms in the pupil plane (pupil tracking mode).
The field orientation on the detector is therefore changing with time, something not well managed by the keyword in the data headers. 2) Uncertainty on the central wavelength due to the spectral type of the target. 3) Uncertainty on the pupil diameter inside the camera filter wheel. These sources of errors are estimated to limit the determination of the relative positions to a few percents. It is expected that pupil tracking will be available by the time the mode is offered. Additional results will be published in the NaCo Web pages in the near future.
HD39213 in K
AB Dor was an excellent commissioning target since it allowed a validation of the technique with respect to the coronagraphic results. However, it is not an excellent target to demonstrate the possibilities offered by aperture masking. This technique is more adapted for imaging details that are within the speckle noise range. Typically, it shows an important advantage for high dynamic range imaging close to the main star (1 to 5 λ/d). We therefore observed HD 39213. This star has a close companion with a flux ratio of the order of a few percent, and an angular separation of less than 100 mas. HD39213 was observed from 2h22 to 3h12 UT. The calibrator, HD 39545, was observed between 2h48 and 3h12UT. H band and K band data consist of 2 data cubes of 24 images of 8 seconds exposure time. Due to a longer integration time, and because the seeing decreased notably (averaging 1.8 arcsec), H band data was mostly useless. We were nevertheless able to retrieve useful information from the K band data by careful selection of images within the data cubes. In fact, it is quite impressive to see how resistant aperture masking is to bad seeing: the likelihood map presented in Figure 4 pinpoint precisely the position of the companion. We recorded an angular separation of 59±8 mas, with a flux ratio of 1.22±0.19%.
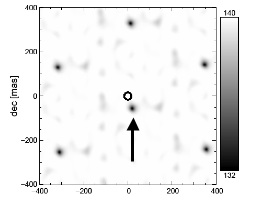
Figure 4:Likelihood as a function of the position of a secondary star.
At maximum likelihood, the flux ratio between the main star and its companion is 1.22±0.19% (arrow).
U-V coverage
This section contains information on the physical dimensions of the sparse aperture masks placed in the Conica camera. These values are necessary to compute the u-v coverage of the instrument.Assumptions:
- The pupil diameter in the camera is 10 mm.
- The clear aperture of the telescope is assumed 8.00m
- The central obscuration assumed 1.116 m.
- Telescope mirror area = 49.29 m
Masks manufactured to fit within slots in the pupil wheel 20 mm outer diameter. Each mask is embossed with an identifier and in addition has orientation marks "+" at the center and towards the edge. Material: .02mm steel sheet. The data for each mask can be found in the u-v coverage datasheet
PSF and MTF
The following images (Figure 5.) were obtained using an internal calibration source and filter IB_2.24.
![]() |
![]() |
![]() |
![]() |
![]() |
![]() |
![]() |
![]() |